Carbon, a fundamental element, forms the basis of countless materials, including diamonds and graphite. Its versatility extends to forming carbides, compounds with distinct properties.
This blog post explores the differences between carbon and its various allotropes and carbides. We will delve into their characteristics, including hardness, thermal stability, electrical conductivity, chemical reactivity, and magnetic properties, providing a comprehensive comparison for industry professionals.
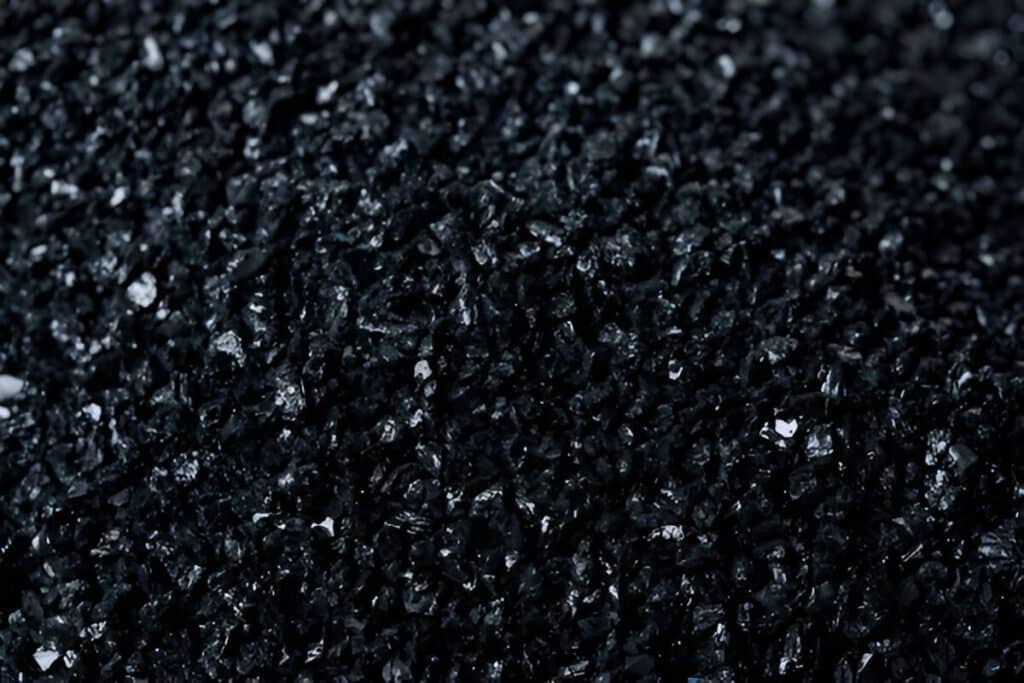
What is Carbon
Carbon is a non-metallic chemical element with the symbol C and atomic number 6. It is the fourth most abundant element in the universe by mass after hydrogen, helium, and oxygen. Carbon is highly versatile due to its ability to form stable covalent bonds with itself and other elements, resulting in a vast array of compounds.
Allotropes of Carbon
Diamond
Diamond is a metastable allotrope of carbon where each carbon atom is covalently bonded to four others in a tetrahedron lattice arrangement, resulting in a highly rigid crystalline structure.
Graphite
Graphite is the most stable form of carbon under standard conditions. It has a layered, planar structure where each carbon atom is covalently bonded to three others in a hexagonal lattice, forming sheets called graphene.
Fullerenes and Nanotubes
Fullerenes are allotropes composed of carbon atoms arranged in a closed or partially closed mesh, typically in the shape of a hollow sphere, ellipsoid, or tube. The first discovered and most famous fullerene is buckminsterfullerene (C₆₀), a hollow spherical molecule resembling a soccer ball.
Carbon nanotubes are cylindrical fullerenes with aspect ratios often exceeding 1,000,000. They can be single-walled with a diameter close to 1 nm or multi-walled consisting of multiple concentric tubes.
What are Carbides
Carbides are compounds composed of carbon and a less electronegative element, typically a metal. In carbides, carbon generally exhibits negative oxidation states, often -4. The relatively small size of carbon atoms allows for extensive insertion into the crystal lattices of metals.
Carbides are characterized by high hardness, high melting points, and good thermal and electrical conductivities compared to the parent metal. These properties arise from the strong bonding between carbon and metal atoms.
Types of Carbides
Carbides can be broadly classified into three categories based on the nature of their bonding: ionic (salt-like), covalent, and interstitial (metallic).
Ionic (Salt-like) Carbides
Ionic or salt-like carbides form between carbon and highly electropositive elements such as alkali and alkaline earth metals. The bonding is characterized by the transfer of electrons from the metal to carbon, resulting in ions held together by electrostatic forces. Examples include:
- Calcium carbide (CaC₂): Used in the production of acetylene and calcium cyanamide. Reacts with water to produce acetylene gas.
- Magnesium carbide (Mg₂C₃): Also used in the production of acetylene. Reacts with water more vigorously than CaC₂.
Ionic carbides are typically unstable and reactive due to the high negative charge on the carbon atoms. They decompose in water and dilute acids to produce hydrocarbons.
Covalent Carbides
Covalent carbides form between carbon and less electropositive elements with similar electronegativities, such as silicon, boron, and tungsten. The bonding involves the sharing of electrons in a covalent manner, resulting in compounds with a high degree of covalent character. Examples are:
- Silicon carbide (SiC): Known as carborundum, it is used as an abrasive and in high-temperature ceramics due to its hardness and thermal stability.
- Boron carbide (B₄C): One of the hardest known materials, used in tank armor, bulletproof vests, and abrasives.
- Tungsten carbide (WC): Used in cutting tools, abrasives, and wear-resistant parts due to its high hardness and toughness.
Covalent carbides generally have high melting points, hardness, and chemical stability. They are semiconductors or insulators due to the absence of free electrons.
Interstitial (Metallic) Carbides
Interstitial or metallic carbides form when carbon atoms fit into the interstices of a metal lattice, often of transition metals. The small size of carbon allows for extensive insertion without significantly disturbing the metal lattice. Examples include:
- Iron carbide (Fe₃C), known as cementite, is a key component in steels and cast irons, contributing to their hardness.
- Titanium carbide (TiC) is used in cutting tools and wear-resistant coatings due to its high hardness and thermal stability.
- Tungsten carbide (WC) is also an interstitial carbide, despite having covalent bonding characteristics.
Interstitial carbides exhibit mixed bonding, with a combination of covalent, ionic, and metallic components. They are characterized by high hardness, high melting points, and good electrical and thermal conductivities.
What are the main differences between ionic, interstitial, and covalent carbides
The main differences between ionic, interstitial, and covalent carbides lie in their bonding nature, which dictates their structure and properties:
Bonding
- Ionic carbides have electron transfer from the metal to carbon, resulting in electrostatic attraction between ions.
- Covalent carbides have electron sharing between carbon and the other element, forming directional covalent bonds.
- Interstitial carbides have a mix of covalent, ionic, and metallic bonding, with carbon atoms inserted into the metal lattice.
Structure
- Ionic carbides have a salt-like structure with alternating cations and anions.
- Covalent carbides have a network covalent structure with strong directional bonds.
- Interstitial carbides maintain the crystal structure of the parent metal with carbon in interstitial sites.
Stability
- Ionic carbides are generally unstable and reactive, decomposing in water and dilute acids.
- Covalent carbides are chemically stable and inert due to strong covalent bonding.
- Interstitial carbides have moderate to good chemical stability.
Electronic Properties
- Ionic carbides are insulators due to the absence of free electrons.
- Covalent carbides are semiconductors or insulators depending on their bandgap.
- Interstitial carbides are metallic conductors due to the presence of free electrons.
Physical Properties
- Ionic carbides have lower hardness and melting points compared to covalent and interstitial carbides.
- Covalent carbides have very high hardness, high melting points, and low electrical and thermal conductivities.
- Interstitial carbides have high hardness, high melting points, and good electrical and thermal conductivities.
Difference between Carbon and Carbides
Hardness and Wear Resistance
Pure carbon, in the form of graphite, has a relatively low hardness of 0.5-1 on the Mohs scale. In contrast, carbides generally exhibit much higher hardness values. For instance, tungsten carbide (WC) has a hardness of 9-9.5 on the Mohs scale, making it one of the hardest materials known.
Thermal Stability and Melting Points
Graphite, the most stable form of carbon under standard conditions, sublimes at 3,642°C (6,588°F) without melting. On the other hand, carbides generally have high melting points but lower than that of graphite. For example, titanium carbide (TiC) has a melting point of 3,160°C (5,720°F), while silicon carbide (SiC) melts at 2,730°C (4,946°F).
Electrical Conductivity
Graphite is an excellent electrical conductor due to its delocalized π-electrons, which allow for easy electron movement. Its electrical conductivity is approximately 3 x 10^5 S/m. In contrast, most carbides are semiconductors or insulators. For instance, silicon carbide (SiC) has an electrical conductivity of around 10^-6 to 10^3 S/m.
Chemical Reactivity
Carbon, particularly in the form of graphite, is relatively inert and resistant to chemical attack at room temperature. However, it can oxidize at high temperatures, forming carbon dioxide. Carbides, on the other hand, have varying chemical reactivities depending on their type. Salt-like carbides, such as calcium carbide (CaC2), are highly reactive with water, producing flammable acetylene gas. Covalent carbides, like silicon carbide (SiC), are generally more chemically inert and resistant to oxidation due to the strong covalent bonding between carbon and silicon atoms.
Magnetic Properties
Pure carbon, in all its allotropic forms, is diamagnetic, meaning it exhibits weak repulsion in the presence of an external magnetic field. In contrast, some carbides, particularly transition metal carbides, can exhibit magnetic properties. For example, iron carbide (Fe3C), also known as cementite, is ferromagnetic, meaning it can be magnetized and attracted to magnets. This property arises from the presence of iron atoms in the carbide structure.
FAQs
How are carbides synthesized?
Carbides are typically synthesized through high-temperature reactions. Common methods include direct reaction of an element with carbon, or the reaction of a metal oxide with carbon in an electric arc furnace, like in the production of calcium carbide (CaC2) from lime (CaO) and coke at around 2000°C.
Can carbides be recycled?
Yes, many carbides can be recycled. Tungsten carbide, for instance, is often recycled due to tungsten’s high value and scarcity. The recycling process involves chemical treatments or zinc melting to recover tungsten and cobalt from cemented carbide scrap, resulting in over 80% material recovery.
Can carbon and carbides be used together in the same application?
Absolutely. In composite materials, carbon fibers are often combined with carbide coatings to enhance properties. For example, carbon fiber-reinforced silicon carbide (C/SiC) composites are used in high-performance brake discs, benefiting from carbon’s strength and silicon carbide’s wear resistance.
Is Carbon or Carbide Stronger?
Carbide is generally stronger than carbon. Carbide compounds like tungsten carbide have extremely high hardness (9-9.5 on the Mohs scale vs 7-8 for carbon steel) and compressive strength (around 530,000 psi for tungsten carbide vs 36,000-65,000 psi for carbon steel). The strong covalent bonds between carbon and metal atoms in carbides make them harder and more wear-resistant than pure carbon materials.